Without question, the United States has become increasingly reliant on space. Both economically and militarily, our dependence on space assets is undeniable. Orbiting satellites provide myriad services that we have become dependent on, such as precise position, navigation, and timing (PNT); communications; weather data; missile warning; and intelligence, surveillance, and reconnaissance (ISR).
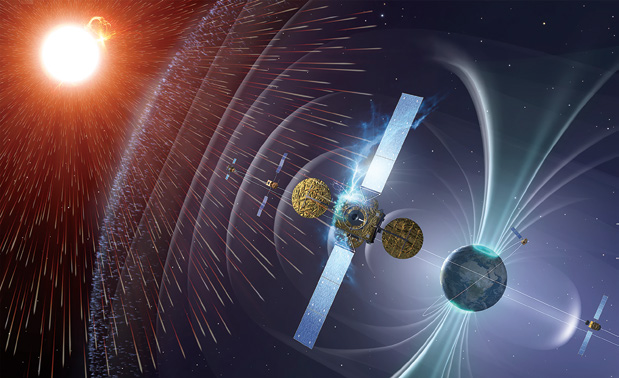
Space Situational Awareness: Space Weather Image credit: European Space Agency / ESA—P.Carril
These functions have served not only as the lifeblood of the global economy over the last decade but also as key enablers in conducting the global war on terror. As the strategic focus shifts to the Pacific region, our reliance on space assets will become even more important, and preserving US space capabilities will prove critical to ensuring America’s military dominance in any future conflict.1
After the Cold War, space became a sanctuary for the United States, which enjoyed almost complete freedom to operate within its vast realm. That situation is certainly changing, however, as many new players enter the space arena and as China begins to emerge as a near peer in space. With China integrating its military and civilian space endeavors and Russia investing in a revival of its space capabilities, both countries present challenges for the United States that we must address.2
Although Iran and North Korea have less mature space programs, their continued intercontinental ballistic missile (ICBM) development efforts and attempts to launch satellites indicate a desire to establish a larger presence in space.3 In addition to developing space-based communications platforms, PNT, and ISR systems, Russia, North Korea, and Iran are working to acquire systems that would effectively deny the U.S. military’s use of space by jamming Global Positioning System (GPS) satellites and other key communications links.4
Imagine a conflict in today’s high-tech warfare environment without the ability to drop GPS-guided munitions, offer persistent ISR coverage via remotely piloted vehicles over the battlefield, or detect the launch of a Scud missile. How would a conflict change if weather information over the battlefield were not available or if we had neither strategic communications nor missile warning during a hostile ICBM launch? It is vital that we preserve space-based capabilities critical to the projection of both air power and sea-based power in the contested and congested space environment projected by the latest national threat assessment.5
If we wish to maintain our superiority in space, we must first have a clear picture of the environment around our space assets and be able to detect any change or potential threat—in other words, we need space situational awareness (SSA). Historically, our efforts to protect US space-based capabilities have relied upon SSA focused on spaceflight safety, a mission that entails the creation and maintenance of a catalog of orbiting satellites, spent rocket bodies, and other debris used to predict and avoid potential collisions in space.
This critical mission reduces the risk to our satellite launches and protects orbiting space assets (both manned and unmanned), all in an attempt to avoid a conjunction between orbiting objects. In addition to destroying the hit satellite (resulting in loss of mission and the significant investment to deploy it), a collision in space—which can occur at speeds up to 17,000 miles per hour—has the potential to produce a large debris field and render an orbit regime unusable. Even though spaceflight safety is vitally important, an SSA concept of operations focused solely on collision avoidance does not do enough to combat the increasingly contentious environment, which includes anti-satellite (ASAT) weapons, communications jammers, and sensor dazzlers.6 The sobering bottom line is that the SSA concept of operations we have relied upon for decades can no longer sufficiently protect our crown jewels in space.7
This article stresses the necessity of maintaining robust SSA, arguing that, to do so, we must pivot from the traditional SSA that emphasizes catalog maintenance to a more tactical, predictive, and intelligence-driven SSA directed by an integrated Battle Management Command, Control, and Communications (BMC3) infrastructure. We must build a new space superiority enterprise around SSA sensors that utilize common data models to support rapid tasking, processing, exploitation, and dissemination across multiple classification levels. It must incorporate tactical intelligence to ensure timely characterization and identification of threats and include a robust set of executable space-control BMC3 courses of action that, given enough warning, we could use to mitigate a threat.
Space Race Revisited
1950s–1970s: The Dawn Of Space
From the earliest days of armed conflict, military forces have endeavored to occupy the high ground of the battlefield, whether a hill, a mountain, the air, or space. Possessing the high ground has always given a military force the advantage over its adversary, regardless of the technologies or strategies of the time. With the advent of the airplane at the beginning of the twentieth century, air became the new high ground and air superiority the rallying cry.
Since the mid-to-late 1950s, technology has advanced to the point where space has become the ultimate high ground. As the Cold War ramped up, the American and Soviet militaries—building upon technologies originally developed to deliver ICBM-carried nuclear weaponry— launched both communications and spy satellites at the same time they built stove-piped command and control (C2) systems.8 Space was an enabler at this point; weather satellites, communications relays, and the earliest spy satellites did not gain further significant utility until the Vietnam War.
1970s–1990: Buildup of Contested Space
During the height of the Cold War, both the United States and USSR developed and tested several ASAT weapons in an effort to gain the ability to hold adversary space assets at risk—to control space. The Soviet Union worked on a co-orbital satellite destroyer or “Istrebitel Sputnikov” throughout much of the cold war.9 One of the most well known ASAT tests involved the direct-ascent ASAT destruction of an experimental satellite nearing the end of its operational life (the Solwind P78-1) by a US Air Force ASM-135 missile launched from a specially modified F-15 on 13 September 1985. The fighter launched the ASAT missile from a location 200 miles west of Vandenberg AFB, California, to its target 345 miles above in low Earth orbit. The 30-pound miniature homing vehicle successfully destroyed the 2,000-pound satellite, producing minimal debris, thanks to its relatively small size and low orbit. The remaining pieces of the satellite then burned up as they reentered the atmosphere. This would be the last ASAT test conducted for another two decades.10
1990s–2007: America’s Growing Dependence on Space
Our experience in Desert Storm was a watershed for space power. . . . Space is now so integral to joint and combined military operations that were we to remove space assets from our military arsenal . . . we would be relegated to employing warfighting tactics much like those of World War II. —General Charles A. Horner, USAF, Retired
The United States conducted a massive integration of space into the American way of war during the decade between the 1991 Gulf War and the wars in Afghanistan and Iraq of 2001 and 2003, respectively. During Operation Desert Storm, which some have called the first “space war,” the breadth and scale of the utilization of space had increased significantly since the Vietnam War, both militarily and commercially.11
The Blue Space Order of Battle (assets used in the execution of the operation plan) included 51 military and 12 commercial satellites.12 Every space mission played a part in Desert Storm (which, by all accounts, involved the greatest deployment of satellite ground stations and pieces of user equipment in history), with each providing a significant edge to the war fighter on the ground.
Even so, we had not yet fully integrated space into our concept of operations—we did not yet have GPS-guided precision munitions, robust satellite communication devices, and tactical ISR at the forward edge of the battlespace. Yet, our forces understood the edge that space systems could provide. A good example is the emergency procurement of early commercial GPS receivers, which were “duct-taped” into helicopters to aid in navigation. Just a decade later in Operations Enduring Freedom and Iraqi Freedom, we used B-52s for close air support missions called in via satellite communications by special operations troops on horseback using laser range finders integrated with the ubiquitous GPS receivers to direct munitions to a precise “danger close” point.13
The Importance of Space Control
Admiral Alfred Thayer Mahan, one of America’s foremost naval strategists, viewed Earth’s oceans as a medium for force projection and commerce, which, when controlled by the appropriate strategies, policies, and doctrine, could provide a nation an advantage in economic and military terms.14 In a similar vein, our nation’s growing use of and dependence on space necessitated the development of effective policies and doctrine, as well as the tools and resources to ensure our effective and proper use of space.
Taking it a step further, Admiral Mahan advocated the principle of “sea control” for the unfettered use of the oceans for a nation’s purposes, an idea that directly translates to the concept of “space control.” To gain superiority in space, the space control mission needed to address not only the surveillance of space but also the protection of US and friendly space systems used for battle management, communications, and intelligence, and the prevention of an adversary’s ability to use space systems and services for purposes hostile to US national security interests. In 1979 the Space Defense Operations Center (SPADOC, later the Space Control Center) was established at Cheyenne Mountain, Colorado, to command and control the space surveillance network, followed by the establishment of Air Force Space Command in 1982 and the unified US Space Command in 1985. For the first time, space was viewed as a theater of operations, and many of the space control systems we are dependent on today had their genesis during those years.15
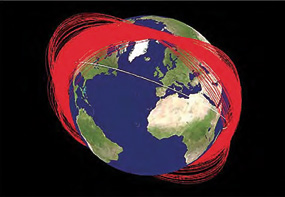
Figure 1. Representation of debris from the Fengyun-1C Chinese weather satellite. (From National Aeronautics and Space Administration, “United Nations Adopts Space Debris Mitigation Guidelines,” Orbital Debris Quarterly News, April 2007, 2, http://orbitaldebris.jsc.nasa.gov/newsletter/pdfs/
ODQNv11i2.pdf.)
Soon after the first “space war” and with the demise of the Soviet Union, Russia bowed out of the space race, and the United States effectively ceased major upgrades to its space control enterprise. The SPADOC at Cheyenne Mountain received only a few minor upgrades in the years after Desert Storm. The SPADOC computer system, which still operates, will remain in its current state until a modernized replacement— the Joint Space Operations Center (JSpOC) Mission System (JMS)—comes online in 2016.
In the years after Desert Storm, America’s reliance on space-based platforms became an ingrained part of our military through the use of precision-guided munitions as well as ship and aircraft navigation. We repurposed satellites originally designed for more basic objectives as global communications relays for commanding remotely piloted vehicles or platforms that relayed ISR data to the war fighter in the air, on the ground, and at sea. This dependence also permeated our civil culture, with space applications becoming part of the shipping, banking, agriculture, and entertainment industries. The loss of GPS alone would have an impact of more than $96 billion per year.16 The satellite industry flourished as the world found new ways to use space systems— both on the military front and in the commercial sector. Faced with few challenges to our superiority in space, we rested on our laurels and enjoyed the unimpeded benefits of a burgeoning space industry.
2007–Present: Space Control at a Crossroads
On 11 January 2007, China changed the status quo in space warfare by firing an SC-19 direct-ascent ASAT missile at its own weather satellite— the Fengyun-1C.17 The kinetic-kill vehicle, a modified version of China’s DF-21 medium-range ballistic missile, and launcher system engaged the satellite at a closing velocity of approximately 17,000 miles per hour at an altitude of 537 miles—200 miles higher than the US ASAT test in 1985.
Unlike the results of the 1985 US test, destruction of the refrigerator-sized Fengyun-1C created a sizable debris field—the largest in history. With that one test, the space catalog grew by over 15,000 debris particles trackable by the space surveillance network (SSN) and the JSpOC and hundreds of thousands of debris particles too small to be tracked by the SSN but still large enough to be a safety concern for human space activities in low Earth orbit.18 Figure 1 below illustrates the extent of the debris field created by destruction of the Fengyun-1C.
The satellite collision between the American Iridium 33 and the Russian Kosmos 2251 communications satellites over Siberia in 2009 was the first publicly confirmed hypervelocity accident between two intact artificial satellites in Earth orbit.19 NASA estimated that the satellite collision created 1,000 pieces of debris, each larger than 10 centimeters (cm) (four inches). The debris field continued to grow, and by July 2011 the SSN had cataloged more than 2,000 large fragments of debris. NASA determined that the field presented only a low risk to the International Space Station, which was orbiting approximately 430 kilometers (270 miles) below the collision course, and to the next shuttle launch (STS-119), planned for late February 2009.
However, to this day, NASA assesses the potential for a collision with this debris field prior to every space launch. As recently as 22 January 2013, a piece of the Fengyun-1C from the 2007 ASAT test is believed to have hit a Russian experimental Ball Lens in the Space (BLITS) satellite, knocking it out of its useful orbit.20 In light of America’s unquestioned dependence on its space assets, it is absolutely critical to the nation that we gain and maintain SSA—to detect, track, and identify orbiting assets as well as any other threat to our systems and to perform conjunction assessments within a time frame and with sufficient certainty to take action to avoid any threat, whether accidental or intentional.
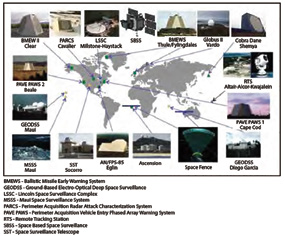
Figure 2. Space surveillance network (2012) and notional additions
Space Superiority Enterprise
Current SSA Concept of Operations: Routine Catalog Maintenance
At present, the SSA mission focuses on the ability to view, understand, and predict the physical location of natural and man-made objects in orbit around Earth with the objective of avoiding collisions. The Secure World Foundation reports 450 operational satellites and more than 10,000 pieces of trackable debris at low Earth orbit, 55 operational satellites and over 500 pieces of trackable debris at medium Earth orbit, and 400 operational satellites and in excess of 1,000 pieces of trackable debris at geostationary orbit. The JSpOC has the best orbital tracking network in the world with a catalog of more than 21,000 resident space objects greater than 10 cm in size.21 However, we must also contend with at least 500,000 bits of debris of 1–10 cm and another several hundred million bits smaller than 1 cm. Moving at orbital velocities of thousands of miles per hour, any of these objects could pose a threat as more manned and unmanned spacecraft are launched and exposed to a debris field growing at an alarming rate. This problem affects the United States and all other spacefaring entities—both government and commercial.
We track the location of satellites and space debris with a collection of radars and telescopes (see Figure 2), many of which are quite old and were not built with SSA as a primary mission. Ground radars such as Globus II, Millstone/Haystack, ALTAIR/TRADEX, the Ballistic Missile Early Warning System, the Perimeter Acquisition Vehicle Entry Phased Array Warning System, and the Perimeter Acquisition Radar Attack Characterization System all have a pedigree in missile warning from the days when the SPADOC was located within Cheyenne Mountain.
Originally, the US Army built and operated the ALTAIR radar between 1968 and 1970 at the Reagan Test Site on Kwajalein Atoll to simulate Soviet radar capabilities.22 Over time, it became apparent that radars could be repurposed or dual-purposed for the SSA mission. Much of our current SSA network is built upon cooperative agreements between government entities in order to fully leverage systems that support multiple missions. Several efforts are in progress to expand this cooperation to global partners, including friendly nations and
commercial entities, as a means of increasing our efficiency in monitoring the global space environment. One such example, the new S-Band Space Fence, is scheduled to come online in 2017 and will assume a critical role within the SSA network.23
In addition to ground radars, optical systems are essential contributors to the SSA mission. The Ground-Based Electro-Optical Deep Space Surveillance (GEODSS) system, which has operational sites in New Mexico, Hawaii, and Diego Garcia, can track objects as small as a basketball more than 20,000 miles away in space.
The GEODSS plays a vital role in tracking space objects, particularly those in deep space. More than 1,200 objects are in deep space in medium Earth orbit, geostationary orbit (GEO), and highly elliptical orbits. At GEO are the vitally important strategic and wideband communications and missile warning satellites.
The Space Surveillance Telescope—an advanced ground-based optical instrument—can search an area in space the size of the United States in seconds and can scan the geostationary orbit belt multiple times per night. It has a field of view three times better than that of the most capable GEODSS, and each night the telescope captures more than 1 terabyte (1 billion bytes) of data. Given this large amount, it is important that we have adequate capabilities on the ground to process and use the information. In the very near future, as new radars and optical sensors come online, the JMS will be the glue that binds the new and legacy capabilities, allowing us to best use the data they provide.
Ground-based radar and optical systems are the workhorses of the SSN for characterizing objects in space, but they are limited by weather, solar blind spots, and their geographical location on Earth. In order to augment these limitations and exploit the ultimate high ground of space, the United States launched the Space Based Space Surveillance System in 2010. The most capable of the SSN sensors, this system provides high capacity and agility, collecting day or night above the weather and improving revisit rates of objects. Ground optical, ground radar, and space optical systems provide a critical contribution to achieving SSA, but each has inherent limitations. Thus, the United States must have all three components in order to gain and maintain robust SSA. Given our dependence on space, it is imperative that we effectively resource and utilize our SSA sensor network to provide the knowledge we need to enable the safe operation of our on-orbit fleet.
Future SSA: Rapid Characterization of Emerging Threats
Because of emerging threats, the SSA mission must move beyond routine catalog maintenance towards a predictive, time-critical BMC3 environment. The Defense Intelligence Agency’s national threat assessments to the Senate Armed Services Committee in 2012 and 2013 both cited China’s growing, increasingly capable military space efforts.24
In its 2013 Annual Report to Congress, which detailed China’s military developments, the Office of the Secretary of Defense highlighted that country’s “multi-dimensional program to improve its capabilities to limit or prevent the use of space-based assets by adversaries during times of crisis or conflict.”25 From the counterspace perspective, Russia and China continue to develop systems and technologies that can interfere with or disable vital US space-based navigation, communications, and intelligence-collection satellites. North Korea has mounted Soviet-made jamming devices on vehicles near the North-South demarcation line that can disturb GPS signals within a radius of 50–100 kilometers. Reportedly, it is also developing an indigenous GPS jammer with an extended range of more than 100 kilometers. Other state and non-state actors rely on denial and deception techniques to defeat space-based imagery collection, conduct electronic warfare or signal jamming, and possibly attack ground sites for space assets.26 It is critical that the United States ensure the capability to rapidly understand when and where its space systems are compromised.
Today’s Command and Control: Modernizing the Space Defense Operations Center
The JMS program, the cornerstone of the space superiority enterprise, will replace the 1980s-era SPADOC system as the C2 system that focuses on planning and executing US Strategic Command’s joint functional component command for the space mission. Unlike other AOC systems, the JSpOC has specialized C2, SSA, and ISR capabilities in support of space control that make inroads into many mission areas.
The JSpOC can be thought of as a combination air traffic control center and AOC but with a span of control extending 22,236 miles outwards into space. For the sake of comparison, an air traffic controller in a tower is responsible for aircraft flying within a 200 nautical mile range of the tower and up to 10,000 feet in altitude—an effective volume of 315,000 cubic miles. Between Earth and the Geostationary Belt, the effective volume of control is 46 trillion cubic miles—about 150 million times as much volume to control! To exacerbate the problem, space offers unique physics limitations, such as a sun-induced blind spot that can render sensitive optics useless for multiple hours per day and vast distances across which electromagnetic waves must travel. Such factors make it difficult to obtain radar returns from which we can glean accurate range measurements and identification of space objects.
To undertake the complex and computationally intensive job of integrating data from our sensor platforms and fusing a useful SSA picture, the current JSpOC operator relies on disparate—in most cases, antiquated—technology platforms such as the SPADOC computer system; Astrodynamics Support Workstation; and Command, Analysis, and Verification of Ephemerides Network (CAVENet).27 Given the growth in the number of spaceborne objects posing a threat to the space systems upon which we so heavily rely, conducting our SSA mission with these legacy systems is not an acceptable way to move forward.
The JMS will replace the legacy SPADOC and ASW processes and capabilities with a modernized, scalable, extensible, and sustainable platform upon which to build the SSA mission set that the United States requires for the twenty-first century. To meet the legacy-replacement goal, the JMS program is developing a government service-oriented architecture (SOA) infrastructure that supports the integration of mission applications while acquiring mature, commercially developed government mission applications. Building the JMS on a robust, disciplined SOA platform is essential to making sure that the JSpOC can evolve over time with new functionalities replacing outdated services and revised software applications integrating new operator-defined tactics, techniques, and procedures. Future capabilities required by the JSpOC after 2015 will call for the development of new applications and procedures as well as the exploitation of new SSA data sources. Further, we can assume that operators will find innovative ways of using the system’s capabilities not imagined when the system was designed— they always do. JMS must enable the JSpOC to exploit this learning.
This is one of the key objectives for the JMS—better, faster, and extensible data integration with a wider variety of data sources. In contrast to the SPADOC system, we expect the JMS to accept and integrate not only traditional SSN tracking data, including information from US missile-warning radars, but also nontraditional formatted observations and ephemerides from a variety of sources, positional data derived from satellite telemetry, and tracking data from foreign sensors. In many cases, the data will be delivered net-centrically, based on work with the Net-Centric Sensors and Data Sources effort, intended to expose such sources.28
Pivoting to Space Battle Management Command, Control, and Communications
The Iridium/Kosmos collision in 2009 and the BLITS conjunction of 2013 remind us that poor SSA is not an option. Moreover, in light of the rising number of spacefaring nations (e.g., China, North Korea, and India), clearly space is becoming more crowded. Because some nations have both stated and demonstrated their intent to attack our dependence on space, we must be able to protect our assets.29 If America intends to maintain its status as the most capable space nation, then—in the face of growing threats—we must evolve our SSA paradigm to do more than just routine catalog maintenance and monitoring of potential conjunctions. Like its AOC counterpart, the space community needs to develop a BMC3 capability that includes AOC-like analysis, data fusion, and identification of threats.
The routine (peacetime) timelines associated with developing our catalog-maintenance-focused SSA are not sufficient to respond to or even anticipate a would-be adversary’s attack in time. Before the joint functional component command for space could start formulating courses of action in response to an on-orbit event—such as a satellite conjunction, debris breakup, or a potential ASAT—decision makers at the satellite operations centers and at the JSpOC would need to develop a timely operational picture of the situation in space. The latter would include all ground assets that can affect objects in space, on sufficient timelines within which to perform observe-orient-decide-act analysis and reach “act” decisions from the appropriate levels in the chain of command—no easy task.
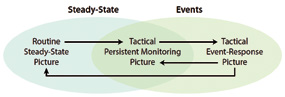
Figure 3. Transitioning the operations tempo of space situational awareness
Elements within Air Force Space Command have begun to examine this issue through “kill-chain” analysis—an in-depth examination of technological needs, materiel solutions, procedural changes, ISR requirements, and concepts of operations necessary for a decision maker to execute a course of action that has been planned for, exercised, and refined by space operators. The kill-chain analysis calls for elements of traditional C2; however, now that space is no longer a sanctuary and response timelines are being compressed more than they have been in the history of space control, our C2 must become even more tightly integrated with communications nodes outside the JSpOC—at the National Air and Space Intelligence Center, National Security Agency, National Reconnaissance Operations Center, or any organization with the requisite space control, ISR, or space warfare expertise.
This modern C2—or C3 when merged with communications—is essential for effective crisis management. The convolution of battle management and C3—BMC3—is what the space superiority enterprise needs in this era of contested, congested, and competitive space control. Space BMC3 goes beyond a routine, steady-state picture, creating a tactical monitoring posture for high-interest items that can affect space assets. With timely indications-and-warning systems, the enterprise can tailor its prepared responses to tactical events without sacrificing its global commitments to catalog maintenance (see Figure 3).
This new space posture demands much more than upgrades to legacy hardware and software. To enhance current operations, the Space Superiority Systems Directorate has teamed with the JSpOC, US Strategic Command, and other combatant commands in designing and exercising new and evolved tactical scenarios. We use collaborative “scrimmages” to extract and exercise exactly how and which parts of currently operational and prototype systems will support a particular crisis scenario. The exercises make use of test-bed and prototype analyst tools, reducing overall execution risk by buying down technical risk, smoothing out integration schedule risks, providing exercise quality feedback on potential real-world performance for prioritization purposes, and opening up tactical-response problems for an expanded community of research-and-development problem solvers.
Despite these great strides in evolving the space superiority mission to enhance its flexibility to change, we must take care to ensure that currently static tasking processes become flexible and adaptive to rapidly evolving threats. These processes need to account for the integration of nontraditional sensors into the JSpOC SOA and the operator’s workspace. Data will be delivered to the JSpOC in various formats and classification levels. The JSpOC SOA platform will interpret and fuse the data, feeding them to space operators and intelligence analysts through a user-defined operating picture at the speed of need—not with the hours or even days of delay with which we currently operate. As the volume of data available to the space operator grows, it becomes more important to rapidly collect, process, and exploit the information, using methods not yet refined.
This capability exists today, but we need to automate it for execution without intervention by the developer or a cadre of engineers. The data will be compatible with various systems through agreed-upon exchange formats and have outputs that can be readily shared among military and civilian operators. Intelligence operators will have to exploit data on the fly through tactical timelines and rapidly disseminate raw intelligence through netcentric means to operators around the world who can interpret information through their user-defined operating picture.
In this new space posture, the roles of some of our existing capabilities will require adjustment. Intelligence assets already play a critical role in characterizing assets in space and focusing indications and warning resources. Although they already lay the groundwork for planning courses of action in space, they are moving into a new role of tactical corroboration and attribution of space events. Take the air world example of foundational intelligence sources discovering information about a potential adversary’s new developmental aircraft. The intelligence community would then use its resources and expertise to determine the capabilities and exquisite features of the new threat.
This foundational intelligence collection is critical and long term, but after the aircraft is produced and enters operations, a different type of intelligence is necessary—one that concentrates on rapid and fleeting collection of very sparse data as an adversary actively tries to avoid detection. The ultimate goal of collectors at this point is not high-fidelity pictures but quick fingerprinting (e.g., which of the threats does this match?). We can do this, but we must emphasize timeliness. A plethora of information systems already makes intelligence assets available. We need to integrate these systems seamlessly into a series of tactics, techniques, and procedures that offer decision-quality information on requisite timeliness.
Future Architecture
To maintain space superiority, we must tightly couple BMC3, SSA, and tactical intelligence in an architecture that enables decision makers to select courses of action in hours rather than days. Today’s BMC3, SSA, and space-control architectures are loosely coupled, but future architectures must have tighter integration. A number of materiel solutions could be integrated into a space superiority architecture. Sequestration hinders our ability to upgrade current capabilities and match those of would-be adversaries; consequently, we must be creative in designing a space superiority architecture for 2020 and beyond.
Like C2 systems in the air world, space superiority BMC3 systems should evolve by leveraging ISR data within federated SOAs and datamining systems. Advances in modern computing make it possible to sort through terabytes of information from many different sources and process these data into actionable information for decision makers. These data must fit within a common data model for exchange among a variety of computing systems. Delivery of the initial SOA in the first increment of JMS, as well as subsequent improvements in future increments, will facilitate cross-domain developments that will allow the JSpOC to connect with AOCs around the world and share BMC3 data at the speed of need.
Air Force and intelligence community agencies have been moving ISR data-fusion centers away from stove-piped systems (e.g., the distributed common ground/surface system [DCGS] and Global Command and Control System [GCCS]) to federated SOAs such as today’s DCGS SOA and the GCCS replacement—Joint Command and Control. Moving to interconnected, federated SOAs allows only a limited opportunity to try out new data-fusion techniques between SOAs.
To address this, the Space Superiority Systems Directorate and the Air Force Research Laboratory are collaborating in building the Action-Centered Rapid Collaborative Application Development Environment (ARCADE) as the key risk-reduction tool for future JSpOC needs. ARCADE will run the most current JSpOC software edition, which will mimic the operational SOA at multiple levels of security and enable commercial developers, other government entities, universities, and so forth, to test and develop applications (using the common data model and software development tool kit) that we could use in future JMS software releases. This collaborative development environment will reduce risk by allowing new technologies to mature before being inserted into the software integration process and hence into the operational SOA, while still allowing testing in an operationally relevant environment. JSpOC operators will gain insight into the ARCADE, and their feedback on candidate upgrades will be a key input to the Requirements and Planning Council—the requirements-setting body within the JMS enterprise.
Through the ARCADE and council process, future JSpOC operators and acquisition leaders in the Space Superiority Systems Directorate can move BMC3 towards a more seamless integration of space and air ISR, giving decision makers the complete, robust, and timely SSA needed in a contested, congested, and competitive space environment.
Conclusion
To maintain space superiority in the face of a changing environment, the United States must find a way to extend the capabilities of current C2, SSA, and ISR systems while investing in new, more capable, but resilient systems to control an increasingly congested environment. More than 1,000 satellites and hundreds of thousands of pieces of debris orbit Earth within an area of 46 trillion cubic miles (see Figure 4). With other nations constantly challenging America’s status as the leader of the pack in space superiority, maintaining a robust suite of ground- and space-based sensors from which to gather data to build our SSA picture is a paramount imperative.
Better protection of our national interests in space demands that we pivot away from the current metric-track catalog maintenance / forensic analysis focus towards a seamless integration of SSA, BMC3, and air/space ISR. The key difference is that C2 implies a mind-set based on a stable, uncontested space environment with hours or even days of response time while BMC3 requires a mind-set of enabling near real time decision making in the face of rapidly changing events that affect national security and America’s trillion-dollar space investment.
Thanks to more capable sensors and C3 systems, the space battle management center of the future will be better at processing future events, more easily upgraded, and able to seamlessly integrate exponential growth in ground, air, and space ISR. Safeguarding US national security satellites depends upon continued support from the entire space community as we work together to operate and counter attempts by China, Russia, Iran, and North Korea to disrupt, deter, and deny our safe and continued access to space—in peacetime and during conflict.30
Notes
1. President Barack Obama (remarks before the Australian Parliament, 17 November 2011)
2. Mark A. Stokes with Dean Cheng, China’s Evolving Space Capabilities: Implications for U.S. Interests (Washington, DC: US-China Economic and Security Review Commission, 26 April 2012)
3. Senate, Annual Threat Assessment, Ronald L. Burgess Jr., Lieutenant General, USA, Director, Defense Intelligence Agency, Statement before the Senate Armed Services Committee, 112th Cong., 2nd sess., 16 February 2012
4. Ibid.; Senate, Annual Threat Assessment, Michael T. Flynn, Lieutenant General, U.S. Army, Director, Defense Intelligence Agency, Statement before the Senate Armed Services Committee, 113th Cong., 1st sess., 18 April 2013,“Russia Delivers Radar Jammers to Iran,” Space Mart: Space Industry News, 25 October 2011, and Patrick Winn, “North Korea’s GPS Jammer Brigade,” Global Post, 16 September 2011
5. Senate, Worldwide Threat Assessment of the US Intelligence Community, James R. Clapper, Director of National Intelligence, Statement for the Record to the Senate Select Committee on Intelligence, 113th Cong., 1st sess., 12 March 2013.
6. Sensor dazzlers are directed-energy weapons intended to temporarily blind or disorient their target with intense, directed radiation.
7. AU-18, Space Primer (Maxwell AFB, AL: Air University Press, September 2009), 68–72, 273–81.
8. “Stove-piped” refers to the design and creation of a system in isolation, without regard to future connectivity or integration with other systems.
9. Anatoly Zak, “Spacecraft: Military; IS [Istrebitel Sputnikov] Anti-satellite System,” Russianspaceweb. com, 24 December 2012.
10. Dr. Raymond L. Puffer, “The Death of a Satellite,” Air Force Flight Test Center Moments in History, 13 September 1985, and Craig Covault, “China’s Asat Test Will Intensify U.S.-Chinese Faceoff in Space,” Aviation Week and Space Technology, 21 January 2007.
11. “Desert Storm: The First Space War,” in Gray Space and the Warfighter, 17 June 1997.
12. Ibid.
13. Maj Michael J. Muolo, Space Handbook: A War Fighter’s Guide to Space, vol. 1 (Maxwell AFB, AL: Air University Press, 1993), chap. 5.
14. AU-18, Space Primer, 137.
15. Benjamin S. Lambeth, “A Short History of Military Space,” Air Force Magazine.
16. Nam D. Pham, PhD, The Economic Benefits of Commercial GPS Use in the U.S. and the Costs of Potential Disruption (Washington, DC: NDP Consulting Group, June 2011), 2.
17. Shirley Kan, China’s Anti-satellite Weapon Test, CRS Report for Congress RS22652 (Washington, DC: Congressional Research Service, 23 April 2007).
18. National Aeronautics and Space Administration, “An Update of the FY-1C, Iridium 33, and Cosmos 2251 Fragments,” Orbital Debris Quarterly News 17, no. 1 (January 2013): 4–5.
19. National Aeronautics and Space Administration, “Satellite Collision Leaves Significant Debris Clouds,” Orbital Debris Quarterly News 13, no. 2 (April 2009): 1–2.
20. Leonard David, “Russian Satellite Hit by Debris from Chinese Anti-satellite Test,” Space.com, 8 March 2013.
21. Brian Weeden, Going Blind: Why America Is on the Verge of Losing Its Situational Awareness in Space and What Can Be Done about It (Broomfield, CO: Secure World Foundation, 10 September 2012), 10.
22. Philip A. Ingwersen and William Z. Lemnios, “Radars for Ballistic Missile Defense Research,” Lincoln Laboratory Journal 12, no. 2 (2000): 245–66.
23. Senate, Space Acquisitions: DoD Faces Challenges in Fully Realizing Benefits of Satellite Acquisition Improvements, Statement of Cristina T. Chaplain, Director, Acquisition and Sourcing Management, before the Subcommittee on Strategic Forces, Committee on Armed Services, 112th Cong., 2nd sess., 21 March 2012.
24. Senate, Annual Threat Assessment, 16 February 2012; and Senate, Annual Threat Assessment, 18 April 2013. September–October 2013 Air & Space Power Journal | 72 Baird The Importance of Maintaining Space Situational Awareness and Taking It to the Next Level Space Focus Feature
25. Office of the Secretary of Defense, Annual Report to Congress: Military and Security Developments Involving the People’s Republic of China, 2013 (Washington, DC: Office of the Secretary of Defense, 2013), 9.
26. Senate, Annual Threat Assessment, 18 April 2013.
27. The heaviest computations are performed on the ASW, a suite of software applications hosted on CAVENet that provides the higher-accuracy satellite catalog needed for a conjunction assessment of spaceflight safety. “Ephemerides” is a table giving the coordinates of a celestial body at a number of specific times during a given period. CAVENet is a legacy system used by the JSpOC consisting of early 1990s-era Silicon Graphics Incorporated workstations and servers. It is an off-line mission support system used for several space surveillance tasks and in-depth analysis.
28. Maj Michael Morton and Mr. Timothy Roberts, “Joint Space Operations Systems (JSpOC) Mission System (JMS)” (presentation, Advanced Maui Optical and Space Surveillance Technologies Conference, 2011).
29. Senate, Annual Threat Assessment, 18 April 2013.
30. Andrea Shalal-Esa, “Pentagon Cites New Drive to Develop Anti-satellite Weapons,” Reuters, 7 May 2013, and Senate, Worldwide Threat Assessment.
About the author
Colonel Mark A. Baird, USAF Colonel Baird (BS, Florida State University; MS, University of Arkansas) is the director of the Space Superiority Systems Directorate at Los Angeles Air Force Base (AFB), California. In this position, he directs the acquisition of space control systems to equip U.S. forces with the capabilities to gain, maintain, and exploit space superiority. He manages a multi-billion-dollar budget, leading a 350-person program office and 1,000-person industry team at multiple locations throughout the country to support operational systems worldwide. Colonel Baird directs the planning, development, testing, deployment, and sustainment of a complex and dynamic portfolio of space superiority capabilities of the highest national priority. He entered active duty in 1989 as a distinguished graduate of the Air Force Reserve Officer Training Corps program at Florida State University. During his career, he has served in a variety of acquisition positions, including contingency contracting officer, procuring contracting officer, program manager, headquarters staff officer, squadron commander, and senior materiel leader. Colonel Baird also has served in fellowships on Capitol Hill and with industry.
Editor’s note
Our thanks to the U.S.A.F.’s Air & Space Power Journal (ASPJ) for allowing us to republish Colonel Baird’s article from their September-October 2013 issue. For more information regarding the ASPJ’s publications, respond to: http://www.air power.au.af.mil